Drugs are exceedingly complicated molecules. The process of getting to know what a drug should look like is, in the words of Edward Holson, Director of Medicinal Chemistry at the Stanley Center for Psychiatric Research, The Broad Institute of MIT and Harvard, “like a chess game.” There is a whole array of factors to consider, whether the pill would survive the digestion journey, whether it would enter the bloodstream, whether it would only target the affected areas, and the list goes on. In the event that a molecule is eventually identified to be suitable as a drug, it would then be ready to undergo large-scale synthesis, except it usually looks like a giant, complicated monster molecule.
What does a chemist do when faced with a task of synthesizing a monster of a molecule? To explain this, I have picked a recent synthesis of the core of oxazolomycin A, a potent antibiotic. Oxazolomycin A is naturally found in bacteria, but like many other drugs, it is usually more reliable and efficient to produce it synthetically in a chemical lab than to extract it from natural sources.

The chemist’s challenge – map out and execute a practically viable chemical synthesis route.
Step 1: Break it up
This is the stage known as retrosynthetic analysis. The chemist looks at the molecule, identifies its weak spots and cuts it at those weak spots into smaller fragments. In this case, the scientists broke up the oxazolomycin target molecule (see picture below) at the points indicated with red squiggles to the fragments separated by the blue squiggles. These fragments, once assembled, can be pieced together directly to form the target molecule.
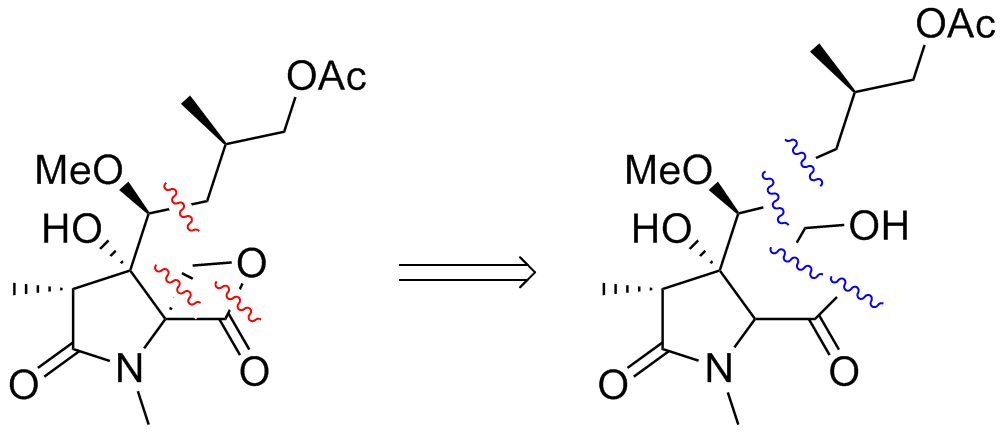
Step 2: What’s in my cabinet?
Then, the chemist looks at what’s available in his/her lab that could lead to the fragments needed to piece the molecule together. Ideally, the chemist could buy these fragments, so that he/she would not waste precious time trying to synthesize them. However, it is more common that the fragments have to be assembled. In this case, the researchers bought the molecule on the right and worked on it until it became the one on the left.

Step 3: Put it together!
First of all, the chemist has to make sure that the right stereo isomers are obtained. As I explained in my previous blog post, stereo isomers are difficult to separate and can cause huge problems if they are not pure. Generally, it should be safe to say that working around stereo isomers is the biggest pain in organic synthesis. In this oxazolomycin target molecule, there are five chiral carbon atoms, indicated in asterisks. The chemist has to get the stereo isomer at each of these five carbon atoms correct, and he/she has to find ways to force the molecule to form each stereo isomer sequentially. In other words, he/she has to prevent getting stuck at a point where the formation of a stereo isomer becomes impossible because there was already another on the molecule.

In this paper, the researchers used an enzyme (enzymes are proteins that speed up reactions in nature and they are all chiral) to install the first chiral carbon atom next to the nitrogen. Then, they cleverly used the existing chiral carbon to force the next three chiral carbon atoms clustered near the nitrogen ring to become the stereo isomer that they wanted. This was done by introducing a really large group on the first chiral carbon to block off one side of the nitrogen ring, so the subsequent reactions could only take place from the other side of the nitrogen ring. The final chiral carbon, further out on the long arm of the nitrogen ring, was made in a similar manner to the first chiral carbon atom, except a chiral catalyst was used instead of an enzyme (a chiral catalyst is like an enzyme, only it is not naturally occurring, it is a molecule made in a lab).
Once the chemist has figured out how the stereo isomers can be obtained, the rest of the molecule building can proceed. The researchers synthesized the oxazolomycin A core in 28 steps and are working to continue the synthesis to the full oxazolomycin A molecule.
28 steps and they were still not at the actual antibiotic!
But this is really the norm in drug development and synthesis. Oftentimes, a pill in the store would have gone through some 30 to 40 synthetic steps and another set of processes for purification and quality control. It is not uncommon to find that by the time the pill is produced, over 95% of the materials used to make it would have gone to waste. Some of us don’t have to think about obtaining drugs to solve common health problems, but in reality, drugs don’t come easy.